Kitabı oku: «All sciences. №6, 2022. International Scientific Journal», sayfa 5
В. English version
1. General concepts of nuclear reactions
By itself, a nuclear reaction is the process of interaction of an atomic nucleus with another nucleus or elementary particle, which may be accompanied by a change in the structure, structure, composition of the nucleus, the formation of new nuclei or elementary particles and the introduction of further changes. Also, the consequence of a nuclear reaction can be its fission, the descent of both elementary particles and massless protons. At the same time, due to the action of several other laws, under which mass can actively be converted into energy and vice versa, the kinetic energy of the resulting particles may well not be equal to the sum of the original ones.
Such nuclear reactions are exo-energetic or energy-releasing. The first nuclear reaction was carried out by Ernest Rutherford in 1917, when alpha particles bombarded the nuclei of nitrogen atoms. It was completely fixed due to the appearance of secondary ionizing particles, whose mileage in the gas was greater than the mileage of alpha particles, after which they were identified as protons. The process was later photographed.
By the way, about this, it is important to say that a Wilson camera is used to photograph nuclear reactions. Speaking about the mechanisms of interaction, we can distinguish two types of such interaction, namely:
1. Reaction with the formation of a composite nucleus, this process consists of two stages, while the incoming particle combines with the bombarded nucleus itself, forming a common core, which later decays. Such a nuclear reaction proceeds at low energies, up to 10 MeV;
2. Direct nuclear reactions that take place immediately, in nuclear time, which is the smallest fractions of a second and are calculated based on other factors, one of which is the time of crossing the nucleus by a particle. Basically, this type of reaction is expressed only at very high energies of the bombarding particles.
If the original nuclei are preserved after the nuclear reaction itself, no new particles are also born, then the reaction is considered elastic scattering in the field of nuclear forces, without any internal interaction. Such a reaction is accompanied only by the transfer of kinetic energy and momentum of one incoming particle to the target nucleus, being called potential scattering and fully obeying the laws of conservation of momentum in this case.
The reaction mechanisms were mentioned earlier, but it is worth dwelling on them in more detail. The first reaction, namely the mechanism of the composite nucleus, was first developed and proposed by Niels Bohr in 1936 together with the famous theory of the droplet model. This theory even today underlies the great ideas about all nuclear reactions.
If we follow this theory, then, as described, the nuclear reaction follows in two stages, while the entire process from collision, formation of a composite nucleus and its decay takes within 10-23-10-21 seconds. And it is important to note that whatever the composite nucleus is, it is always excited due to the excess energy that is introduced by the particle in the face of the binding energy of nucleons in the composite nucleus and part of the kinetic energy of the composite nucleus, which is equal to the sum of the kinetic energy of the target nucleus with a certain large mass number and the incident particle in the system of the so-called the center of inertia.
Here it is important to define such a concept as the excitation energy of a composite nucleus, which was formed during the absorption of a free nucleon. It is the sum of the binding energy of the nucleons of the target nucleus and part of its kinetic energy (1).

Part of the kinetic energy due to the large difference in the masses of the nucleus and the nucleon in such cases becomes equal to the kinetic energy of the bombarding nucleon. On average, the binding energy is equal to 8 MeV and can change only with the distinctive features of the composite nucleus formed in this process, but for the precisely specified target nucleus and nucleon, this value is a constant. The kinetic energy of a particle can be anything, for example, in nuclear reactions where a neutron strikes, due to the fact that there is no repulsive force of the nucleus – the Coulomb barrier, their energy can be extremely close to zero.
Thus, the kinetic energy is the minimum excitation energy of the composite nucleus.
And it is from the statement of the presence of a composite nucleus and the existence of nuclear decay channels that we can conclude about the existence of reaction channels. The reaction channels themselves are the ways of transition from an excited to an unexcited state. The type and quantum state of the incoming particles and nuclei before the start of the reaction determine the input channel of the nuclear reaction, after the completion of the reaction, the totality of the formed particles, that is, the reaction products and their quantum state is determined by the resulting output channel of the reaction. The complete characterization of the nuclear reaction is carried out by input and output channels.
The composite nucleus itself lives for quite a long time, due to which the choice of the reaction channel itself does not depend at all on the method of formation of the composite nucleus, due to which it «forgets» how it was formed. This becomes the reason for the assertion of the independence of the processes of the organization of the composite core and its disintegration. A striking example can be the situation of the formation of an excited aluminum-27 nucleus in the following ways (2).

But it decays violently in the same way in all cases, provided that the excitation energy is the same. But at the same time, there is also a possibility of reverse decay of any of these reactions, with a certain probability that does not depend on the history of the origin of the excited nucleus itself. If we talk about the probability of such events, then the dependence becomes between the grade of the target nucleus and the energies.
As previously indicated, nuclear reactions can also proceed through a direct channel of interaction at high energies, since the nucleons of the nucleus can be considered as free. The difference from the previous composite core model from the direct reaction model initially consists in the distribution of the momentum vectors of the particles-products of the nuclear reaction, relative to the momentum of the bombarding particles. If spherical symmetry operates in the composite model, then in this case the geometry is simpler and the advantage in choosing the directions of the resulting particles is in the direction of the incoming particles.
Earlier, the concept of the probability of a nuclear reaction was mentioned, which is represented by a quantity called the effective cross section of a nuclear reaction. In the laboratory system of the report, the resting situation of the target nucleus is taken, the probability of interaction is determined by the product of the cross section by the flow of incident particles, while the cross section is expressed in units of area, and the flow in the number of particles crossing the unit area per unit time. The cross section of the nuclear reaction itself is calculated in extremely small units of area – barns equal to 10—24 cm2.
The ratio of reaction cases attributed to the number of particles bombarding the target is called the yield of a nuclear reaction. This value is determined experimentally by quantitative measurements, which is associated with the cross—section of reactions, and the measurement of this output is in essence the measurement of the reaction cross-section.
The laws of physics, including conservation laws, of course also apply in nuclear reactions. These laws impose certain restrictions on the possibility of carrying out a nuclear reaction itself. There are also some more specific conservation laws peculiar to the microcosm, an example of such can be the law of conservation of the baryon or lepton number. They are performed on all known reactions, but some other laws of parity conservation, isospin, strangeness, only act in fundamental interactions. The consequence of them is the selection rules that determine the real and impossible nuclear reactions that can be carried out.
The law of conservation of energy in nuclear reactions acts predictably, but very specifically for representatives of the macrocosm. In this case, the equality of the sums of the total energies (3) is fulfilled.

If we paint (3), then we can get (4), from which follows the reaction energy (5), which satisfies (6).



Thus (5) can also be rewritten as (7).
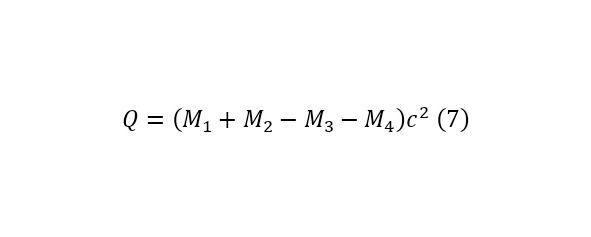
If the reaction yield is greater than zero, then this reaction is exo-energetic and is accompanied by the release of energy into the kinetic energy of the reaction products, in the opposite case – absorption and is called endo-energetic. The adjustment of such a process becomes clear both by the mass difference before and after the reaction, and with a positive difference, we can say that it turns into kinetic energy and the reaction generates energy, in the opposite case, that is, with a negative difference, the process absorbs it.
The law of conservation of momentum also applies, which is very noticeable in direct reactions (8).

At the same time, there is a law of conservation of momentum and a number of other laws, but the most basic ones acting in the reaction are these two conservation laws.
But now it is important to focus on the types of nuclear reactions, and there are several of them: nuclear fission reaction, fusion, thermonuclear reaction and photonuclear reaction. The first type is a nuclear fission reaction, this is the process of splitting an atomic nucleus into two, and less often into three nuclei with close nuclear masses, which are called fission fragments. Other reaction products may also occur, including light nuclei – alpha particles, deuterons, as well as neutrons and gamma quanta. Fission itself is spontaneous and spontaneous, or forced, due to interaction with other particles, for example neutrons. The fission of heavy nuclei is in most cases an exo—energetic process, which makes it possible to obtain energy from radiation and kinetic energy of products from this process.
The nuclear fusion reaction is the second nuclear process, which consists in the fusion of two nuclei to form a new, heavier nucleus. This process is often accompanied by the emission of gamma rays or other elementary particles. Fusion of nuclei is most often an endo-energetic process, which most often requires the introduction of energy through the kinetic energies of particles in order to overcome the Coulomb barrier – the electrostatic repulsion of nuclei. The fusion of two nuclei and giving them energy can be realized, as it is not difficult to guess in charged particle accelerators, or these particles originally possessed this energy, for example, cosmic radiation particles, but there is another way – it is heating matter to extremely high temperatures in a special thermonuclear reactor, where the kinetic energy of particles and temperatures are extremely huge.
In this way, it is possible to approach thermonuclear reactions. In such reactions, the fusion of light nuclei leads to the conversion of the excess mass of the original nuclei into energy, since the total mass of the merged nuclei is greater than the mass of the resulting nucleus-the reaction product.
From this it can be concluded that the nuclear fusion reaction of the initial nuclei must have a relatively large kinetic energy, because they experience a rather powerful electrostatic repulsion when passing from their side of the Coulomb barrier. According to the molecular kinetic theory, their kinetic energy can be represented as the temperature of the entire substance, therefore heating will lead to an increase in the kinetic energy of the composite particles and their fusion. This is how nucleon synthesis develops in the bowels of stars with the formation of new nuclei under enormous temperatures.
In particular, the fusion reaction of protons and helium nuclei occurs in large quantities, and as a side result, other isotopes of substances are formed, including deuterium and tritium, as isotopes of hydrogen. And finally, the last type of nuclear reaction is a photonuclear reaction, in which case a gamma quantum is absorbed with sufficient energy to excite the nucleon composition, that is, the nucleus, so that it becomes composite, that is, it can be considered as such, and also releases a different structure from itself, or decays.
This process is called a photonuclear reaction or a nuclear photoelectric effect. And in conclusion, it is worth noting that nuclear reactions can be written as in the form of an equation, as demonstrated earlier, or, for example, in (9), there is also a slightly different notation (10).
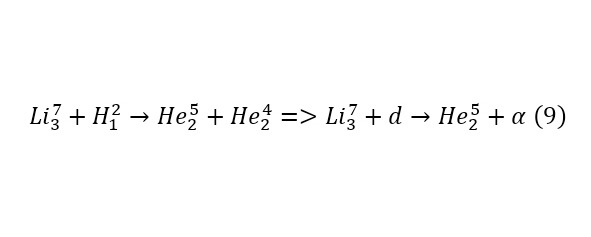
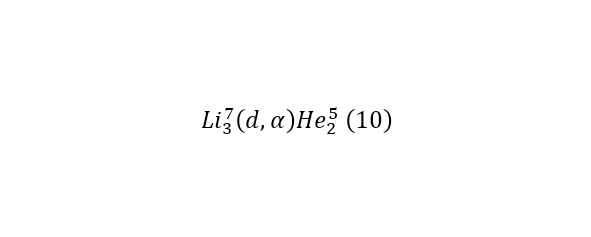
As a result, it can be concluded that it is very important for any researcher in contact with this field, including the physics of resonant nuclear reactions, to have knowledge about the nuclear reactions themselves.
And if you notice, then as it was noted, nuclear physics has been developing for a long time, not to mention the time that humanity has spent to study the structure of all matter and matter as a whole. But active research has led to the very recent discovery of a new direction in this field, namely, the physics of resonant nuclear reactions. For the first time such terminology was used and practically demonstrated in the monograph of 2021 by Aliyev I. H. and Sharofutdinova F. M. «The use of accelerators and the phenomena of collisions of elementary particles with high-order energy to generate electrical energy. The Electron Project», which later received its continuation.
2. Physics of resonant nuclear reactions
The creator of the physics of resonant nuclear reactions is I. H. Aliyev, but what is the basis of this discipline? It studies and determines the most favorable conditions for the occurrence of a kind of power surges of the products of nuclear reactions, which are called resonances. To put it simply, when a nuclear reaction takes place, it forms reaction products, which were discussed in one of the previous lectures, and all the circumstances that lead to the reaction being more efficient and their energies being greater are what the physics of resonant nuclear reactions studies.
Let’s take an example. Let charged particles be directed to the nucleus of some element, it can be protons, ions, electrons, anything. And when approaching the nucleus, the phenomenon of Coulomb repulsion occurs, it acts only on charges of the same name, namely on nuclei, but does not act on neutral particles, for example, neutrons, although the neutrons themselves also have a minimal charge. The particle spends some energy on overcoming the Coulomb barrier and remains with some of its part, which it spends on overcoming the nucleus itself and further passing the nuclear reaction.
The corresponding energy is released in the reaction, if it is endo-energetic, due to the inequality of the masses, that is, some part of the mass is converted into energy and it is already received by the products of the reaction itself – the flying particles, and they also receive the remaining part of the energy from the bombarded particle. And the total energy of these reaction products is determined by the appropriate mathematical apparatus, but we need a power jump.
Power is the product of the beam current by its energy, that is, voltage. The energy is really large for favorable reactions and is measured in MeV, but the current is extremely small. We need to somehow increase it. To do this, it is necessary to understand the phenomenon of the probability of a nuclear reaction. The beam itself is both a wave and a corpuscle, that is, a particle, according to the particle-wave dualism, which you can learn more about from the course of quantum physics, so it has its own de Broglie wavelength (1).
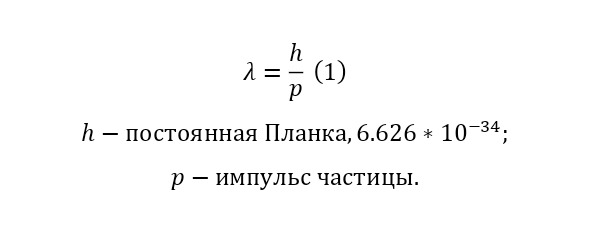
And when a particle approaches the nucleus, even if it did not hit it and did not touch it, if it is at a distance of its wavelength, then there will be an interaction. Yes, indeed, even without touching a particle, it can «hit» and enter into interaction, these are the laws of the microcosm. So, you need to increase this wavelength, and for this you need to reduce the pulse, but to reduce the pulse, you need to reduce the speed.
But it is necessary to reduce the velocity so that the particle passes the Coulomb barrier, from this we can conclude that the energy of the particle should be as close as possible to the Coulomb barrier. And here, the value of the Coulomb barrier is the resonant energy of this nuclear reaction.
Now, how to determine the output power? To do this, you need to calculate the energy, which is already easy to do, but how to determine the resonant current? To define it, imagine the following. The target plate consists of arranged atoms and let a certain number of charged particles enter inside. If we place a reference frame at the beginning of the target, then we can use the following statement that the particles will pass through some part of the target, which begins at a certain coordinate and ends at the coordinate of the sum of this coordinate and the thickness of the part itself, and the thickness is equal to the difference of these coordinates.
The question arises to this condition: how many incoming charged particles will enter into the interaction? To do this, we indicate that there are N (x) particles at the first coordinate, and dN at the end point N (x), respectively, where dN is the number of interacting charged particles.
Let’s determine the number of cores in this segment of two coordinates – x and x+dx, if the thickness between them is dx. To do this, we introduce the value of the density of nuclei, which determines the number of nuclei of a substance per unit volume, it is defined as the ratio of the density of a substance to its atomic mass in kg and changes into a nucleus / m3 (2).
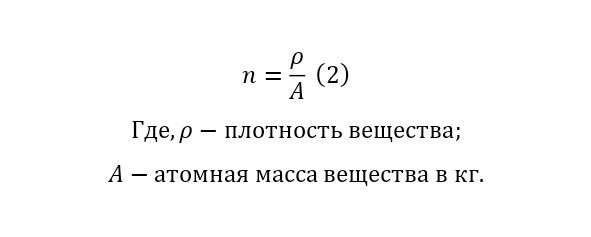
To determine how many cores there are at a specified point, it is enough to multiply this value (2) by the volume in this part of the plate, for this its area is multiplied by the thickness and by (2), which is indicated in (3).

But what is the area, once in which the core will get into the interaction? For one nucleus, we introduce the concept of the nuclear effective cross section, the same region, and since the actions take place in a circle relative to the nucleus of an atom, this value is determined by (4).
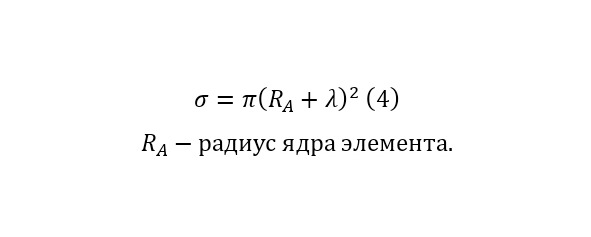
Thus, the area available for interaction is (5).

But the ratio of this area to the entire area of the plate is equal to the ratio of the number of all particles remaining without interaction to the total number of particles, that is, it is true (6).
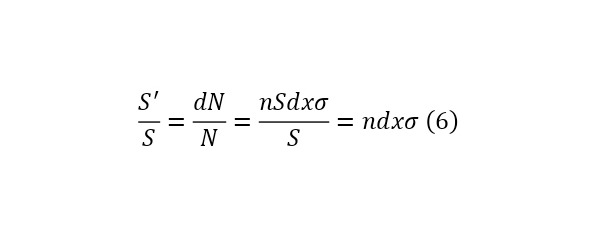
Now, we introduce a numerical definition for (6), and for this we integrate both parts (7) separately into (8) and (9), and then we get the overall result (10).
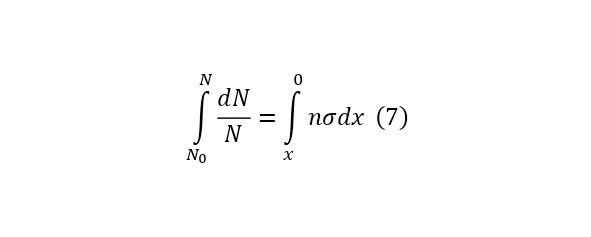



From here we can get the value of the interacting particles (11).
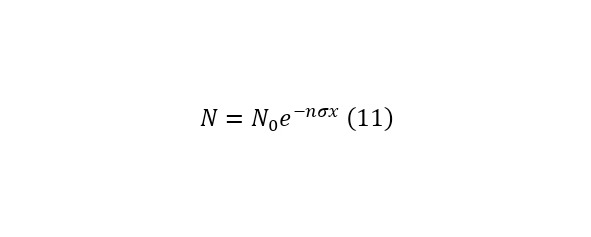
And the output power can also be calculated thanks to (12).

Hence, a jump in power is obtained, that is, a resonance when approaching the energy of the Coulomb interaction in a nuclear reaction. It is this process that is the main one in this direction, which allows for the calibration of energy to receive sharp jumps in power, and in order to implement them, it is necessary to create and develop special monoenergetic accelerators of charged particles with the first linear acceleration, then cyclotron.
Today, the only monoenergetic accelerator in the world is being developed by Electron Laboratory LLC together with the Joint Institute for Nuclear Research and the Federal State Unitary Enterprise Dmitry Vasilyevich Efremov Research Institute of Electrophysical Equipment and other organizations.
To describe the accelerator itself, it is enough to cite a small quotation from the monograph of Aliyev I. H. «New parameters for nuclear reactions for the implementation of charged particle accelerator LCU-EPD-300»:
«When the urgency of the problem of energy starvation on a planetary scale has been proven and demonstrated more than once, the problem of the need to create a device and method for generating electric energy with high efficiency on an extremely large scale, which would allow solving this problem and opening the way for a whole range of numerous projects and scientific works in need of such a source of electric energy, becomes the following a stage in the development of this large project.
And since the necessary research was carried out in the field of searching for such a source and method of energy generation, nuclear reactions were finally recognized as a solution that would increase their own cross-section, therefore, both the probability of passing the reaction itself and the number of active reactions, which of course is directly related to the overall efficiency of the entire nuclear reaction. What follows when taking into account that the energy of the flying particles from the nuclear reaction, in the entire particle picture, is the total voltage, and the number of flying particles, due to their charge, creates a parameter of the current strength of the system.
Due to the fact that the energies are selected in such a way that after passing the Coulomb barrier, the particle has an energy equal to the energy of its thermal counterpart and this fact alone increases the effective cross-section of the entire nuclear reaction into which the particle enters, then such nuclear reactions can be called resonant, due to the fact that they cause resonance in the system and only this they increase the overall efficiency of the entire process.
Resonant nuclear reactions were first discovered in September 2021, after which active research was carried out, which led to a number of publications, the most significant of which was made in December 2021, which is the monograph of Aliyev I. H. and Sharofutdinova F. M. «The use of accelerators and the phenomena of collisions of elementary particles with high-order energy to generate electric energy. The Electron Project», which led to research in the field of searching for this method for 12 years, taking into account that the search in the field of atomic nucleus and elementary particle physics, as well as quantum physics, took place for a significant 5 years. The name of resonant nuclear reactions was given to these systems in January 2022 by Karimov Bokhodir Khoshimovich and appears for the first time in this research.
Due to the fact that the relevance of resonant nuclear reactions quickly follows from the above, it remains to prove the relevance of the fact that a charged particle accelerator, a special type of LCU (Linear Cyclotron Accelerator), its class EPD-20, is necessary for the implementation of these nuclear reactions, it follows from the parameters that proton and deuterium beams are beams in it the Electron project with an energy of up to 20 MeV. Due to the fact that the energy must be selected, for example, for a conventional nuclear lithium-6 bombardment reaction with the release of two alpha particles, it is necessary to have a proton with an energy of 1.613245483 MeV, and only in this case it will be assumed that the final energy of the proton, after passing the Coulomb barrier at the nuclear radius, will be 0.25 eV, due to what does a proton become, what is called «thermal» and the effective cross-section of this nuclear reaction is already measured in huge units – kBn.
But today there is no LCC class accelerator on the whole planet, not to mention a detailed type, having a common LCC-EPD-20 encoding, which could give a proton energy equal to 2,312691131 MeV for the first, 1,978142789 MeV for the second, 1,613245483 MeV for the third and 4,457595117 MeV for the fourth reaction, not because this energy is not achievable, by no means, this energy is scanty in accelerator physics, since modern particle accelerators appear with energies in GeV and TeV. The reason for the difficulty of achieving such results is precisely the accuracy, accelerators can give energy in 1 MeV, 1.5 MeV or 2 MeV, that is, specific values whose accuracy does not exceed 1 or 2 orders of magnitude (by order we mean the order of the fraction or more precisely the negative degree of the base of the exponential function, that is, 10, presented in the module), and as you can see, much greater accuracy is needed for this experiment.
The importance of research on resonant nuclear reactions has been repeatedly stated in a number of scientific articles and ongoing research, and a special monograph «The use of accelerators and the phenomena of collisions of elementary particles with high-order energy for generating electrical energy» was devoted to this. The Electron Project», in which 6 nuclear reactions were described in detail, in 4 of which the process of bombarding a target made of beryllium, boron, aluminum and lithium with protons took place, and in 2 of them, the target was bombarded with lithium-6 and lithium-7 deuterons, due to which they stood out along with the main product reactions were carried out by alpha particles, and also a whole complex of other particles, which, after deviations in the MHD generator, were represented as an electric current.
Speaking about the described scientific work, it is important to note that it was primarily a theoretical work in which calculations of extremely high values took place in connection with the current, when the charges of the beams are extremely large, as are the currents, reaching several kA. And only at the end more approximate data were taken into account. In this case, the calculation is also carried out at the moment when the currents are small and more close to the real ones. For comparison, the currents in the newly created DC-280 cyclotron did not reach a value of 1 A, but were measured only in mA.
The same parameters can be given for the EG-2 SOKOL electrostatic accelerator, now owned by the Research Institute of Semiconductors and Microelectronics at the National University of the Republic of Uzbekistan.
Therefore, in order to carry out this kind of nuclear reactions, when special conditions are necessary, they must once again be specified and clarified, as close as possible to the real values. In addition, if we dwell in detail on the mechanism of reactions, we get a picture from the fact that, as indicated, it is important to have a special device – an accelerator of charged particles, which could impart more energy in the amount of several MeV, for a charged particle. After that, this particle would come across a target of a certain substance, thanks to which a certain nuclear reaction took place. At the same time, a number of processes occur, one of which is overcoming the Coulomb barrier, that is, even if a nuclear reaction occurs with an energy output, the particle must still expend some energy to carry out this action, but if you choose a general combination as follows, so that such an amount of energy is expended, so that eventually a small amount remains by turning the incoming particle into a slow one, the probability of this reaction passing sharply increases to not small values, already after the Coulomb barrier, when Coulomb forces are no longer taken into account and the process takes place at a nuclear radius, as indicated.
Thus, it is important to create an LCU that would give energy to charged particles with a 9—10 order, which significantly increases the efficiency of the entire system under study and leads to a more accurate determination of the Coulomb and other barriers of any reaction. At the same time, this LCC has a number of advantages along with all available accelerators, since, to begin with, it is a combination of two classes of accelerators: cyclic and linear.
Speaking of accelerators, it is important to note that accelerators themselves are simple, in which particles are accelerated by an electric field, the whole principle is based on this. It is also impossible to doubt that the time has finally come for the reaction of the first resonant nuclear reactions at the first LCC. After all, if we resort to history, then, for example, the very first accelerator was built in 1930 by Lawrence Berkeley. The first accelerators are considered to be the accelerators of 1931, when a 23 cm ring cyclotron was created at the University of California to accelerate hydrogen ions with an energy of 1 MeV. A 28 cm ring proton cyclotron with an energy of 1.2 MeV was also developed in Berkeley in 1932. There, at the University of California, Berkeley, a 68 cm ring deuterium cyclotron with an energy of 4.8 MeV was developed from 1932 to 1936; a 94 cm ring deuterium cyclotron with an energy of 8 MeV was developed from 1937 to 1938; a 152 cm ring tritium cyclotron with an energy of 16 MeV was developed from 1939 to the present time; from 1942 to the present The operating time is 467 cm ring cyclotron for various charged particles with an energy of more than 100 MeV. At the same time, in 1932, a proton electrostatic proton accelerator with an energy of 0.7 MeV Cockcroft-Walton was constructed at the Cavendish Laboratory, acting thanks to the voltage multiplier of Ernest Thomas Sinton Walton and Sir John Douglas Cockcroft (winners of 1951), already better known as the Cockcroft-Walton voltage multiplier.
Also known are Harvard accelerators (1949—2002), Oak Ridge National Laboratory (1943-present) for protons and uranium nuclei with energies from 160 MeV. Synchrotrons were also created, known as the cosmotron at Brookhaven National Laboratory, 1953—1968. 72 meters for protons at 3.3 GeV, also the Birmingham sychrotron, Bevatro, the Saturn accelerator, the Russian synchrophasotron in Dubna, the Proton cyclotron at CERN. Listing accelerators can be quite a long process, not to mention describing each one, due to the difference in their types, characteristics and physics. Therefore, there is no room for doubts about the passage of a sufficient path in this area on the part of world science to begin research and work in the design of the newest resonant-type cyclotron.
Ücretsiz ön izlemeyi tamamladınız.